Researching 3 treatment approaches
Gene therapy, RNA technologies, and gene editing are 3 approaches being explored for the treatment of rare genetic conditions. Sarepta research focuses on the potential of each of these approaches to develop products that may impact conditions such as Duchenne muscular dystrophy and the limb-girdle muscular dystrophies.
Exon skipping: making new connections
Exons are segments of genes that are linked together in a string to provide the instructions for making proteins. For example, a large string of 79 exons provides the instructions for making dystrophin, a protein that muscles need to work properly. If a mutation occurs, such as when one or more exons in the string are missing, the body can’t read the genetic instructions to make dystrophin. Without this protein, muscle development and function can be affected.
By “skipping” over certain exons, new connections can be made that enable the body to make a shortened version of the missing or non-functional protein. Exon-skipping therapies are the cornerstone of Sarepta’s current RNA technologies.
Exon deletion tool
A genetic test can reveal a missing exon—known as an exon deletion. If a genetic test reveals an exon deletion, use the Exon deletion tool at Duchenne.com to help prepare for a discussion with your doctor or genetic counselor.
ASOs: targeting single genes
Exon-skipping therapies are sometimes referred to as ASOs (antisense oligonucleotides), which are short molecules that can zero in on specific sections of RNA that may be carrying incorrect instructions to cells. They make up a broad category of investigational products that use synthetic nucleic acid sequences for therapeutic purposes. ASOs can alter RNA and reduce, restore, or modify protein expression through different mechanisms. They are especially promising for rare conditions that are caused by mutations in single genes.
PMOs: researching RNA technology
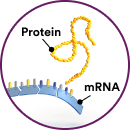
There are multiple FDA-approved PMOs (phosphorodiamidate morpholino oligomers), and additional research is ongoing to investigate others. PMOs are man-made ASO molecules modeled after the natural framework of RNA. A clinician would use the results of a genetic test to determine if a patient is amenable to exon skipping and if a PMO is an option for treatment, because each PMO is specific for a range of deletions that may be found in a gene.